Journal Search Engine
ISSN : 2287-7851(Online)
DOI : https://doi.org/10.11626/KJEB.2013.31.2.165
Distribution Patterns of Calanoid Copepods along the Seomjin River Estuary in Southern Korea during Summer
Abstract
INTRODUCTION
Behavioral adaptations promoting retention within geographical areas seem to be important in marine zooplankton (Kaartvedt 1993; Schlacher and Wooldrige 1995), and such behavior may decrease the actual influence of advection on population dynamics. In particular, migration pattern and retention strategy in tidal estuaries are closely related to the ecological processes of that estuary. Tides determine habitat accessibility for tidal migrating species while the semi-diel cycle actively controls visual conditions and diel changes in organisms. These ecological processes, such as instantaneous variations in salinity caused by tides, increase the mortality rate of early copepod stages. Although copepod position in the water column is greatly affected by water movements such as tides and currents, they are not totally at the mercy of these influences and have been known to migrate vertically and horizontally (Morgan et al. 1997; Kimmerer et al. 1998). However, it is still not fully clear how much of copepod movement is caused by tides and how much is in response to the surrounding physical environment. Although vertical migration has been observed to occur at specific tidal periods (Wooldridge and Erasmus 1980), varying migration patterns are exhibited by different copepod species and even intra-species in different environmental settings, which is more complicated by their species-specific life strategies (Head et al. 1985; Hays et al. 2001). However, tides have often been regarded as playing a constraining role on migration.
Several reports have indicated that, despite their weak power of locomotion, some species of zooplankton occurring in estuaries can actively cope with tidal transportation and remain at their preferred salinity concentrations (Cronin et al. 1962; Wooldridge and Erasmus 1980; Collins and Williams 1981; Hough and Naylor 1991; Kimmerer et al. 1998; Ueda et al. 2004; Park et al. 2005). Moreover, other reports have indicated that the pattern of species appearance in estuaries is related not only to salinity but also to temperature, food, turbidity, tides and other factors (Castel and Veiga 1990; Jerling and Wooldridge 1991; Laprise and Dodson 1994).
In this study we aim to highlight the effect of tide controlling horizontal distribution of some calanoid copepods along the Seomjin River estuary, Korea during the rainy season of August.
MATERIALS AND METHODS
1. Study area
The Seomjin River is 226 km long, making it the ninth-longest river in Korea. The basin area is 4,897 km2, covering southeastern Jeollabuk-do as well as eastern Jeollanam-do and western Gyeongsangnam-do provinces. Water depth in the estuary averages at 7~8 m. Average annual rainfall is 1,253 mm, with over 70% falling in summer. Tides affects to about 18 km in the upper stream from the mouth of the Seomjin River estuary. The sampling stations are showed in Fig. 1.
Fig. 1. Map of the sampling stations in the Seomjin River estuary, Korea.
2. Sampling methods
The field survey was conducted August 9th 2006. All copepods were deep horizontally towed near bottom at the speed of 2 knots from 17 stations during flood and ebb tides along a gradient salinity using a NORPAC net (mesh size 200 μm; mouth diameter 45 cm) during 5 minutes. Distance between each station was about 1 km. Sampling was over within 3 hours starting at each high and low tide using a high speed boat. Water temperature and salinity were measured by 600 MKIII CTD (UK, Valeport) and ACL 1150- DK (Japan, Alec co.).
Abundance of copepods were estimated from rotations of a flow meter (General Oceanics co.) attached to the net mouth. After samples were immediately preserved in a 5% neutralized formalin and estuarine water solution, copepods were identified at a species level and counted using light stereomicroscopes (Nikon) according to their identification characteristics. According to Ekman’s classification system (Day et al. 1989), there are three salinity divisions in the estuarine zone; oligohaline salinity (0~5.0), mesohaline salinity (>5.0~18.0), and polyhaline salinity (>18.0).
Rainfall data from the study area were provided by the meteorological agency (http://www.kma.go.kr/).
3. Statistical analysis
For correlation to detect any significant density differences among the stations at specific tides, and between high tide and low tides, ANOVA and student’s t-test analysis were carried out using SPSS (Version 12). Data were log (X+1)-transformed for the statistical analyses. The density of copepods was calculated by ind.m-3.
RESULTS
1. Tides and salinity
Salinity was in the range of 0.1 to 29.3 for the 17 stations. Salinity of less than 1.0 was found in the upper stream beyond station 5 in high tide, while being noted as far back as station 9 in low tide. Salinity of more than 18.0 was found downstream of station 11 in high tide, reducing to 16.0 in low tide (Fig. 2). In particular, salinity fluctuated strongly during high tide and followed the same pattern in low tide.
Fig. 2. Salinity ranges of in (a) low tide (upper) and (b) high tide (lower) of the Seomjin River estuary.
At both high and low tides, copepod abundance was high at salinity of 20.0~23.0 and low at salinity of <2.0.
2. Copepod populations and their density
In total, ten copepods were recorded from the Seomjin River estuary. Among them the major copepods were Tortanus dextrilobatus, Pseudodiaptomus koreanus, Acartia ohtsukai, Sinocalanus tenellus and Paracalanus parvus s. l., while other minor copepods taxa were Calanus sinicus, Acartia forticrusa, Centropages dorsispinatus, and Labidocera rotunda.
Copepod movement patterns were affected by salinity ranges in estuary zones and their highest density was shown to be polyhaline, which migrated gradually from mesohaline to oligohaline zones. The highest density was observed in mesohaline zone at high tide, while in contrast the lowest density was observed in mesohaline zone at low tide (Fig. 3).
Fig. 3. Abundance of copepods (ind.m-3) in high and low tides according to salinity divisions; e.g. Oligohaline (<5), Mesohaline(5~18) and Polyhaline (>18).
The density of oligohaline species (Pseudodiaptomus koreanus, Tortanus dextrilobatus, and Sinocalanus tenellus) was high in the low salinity estuarine zone during flood/low tides. However, the density of Tortanus dextrilobatus was high at ebb/high tides in the mesohaline zone. Although oligohaline copepods varied highly in density within different salinity zones and sampling locations, their density was widely distributed during ebb tides. During both tides, the densities of Pseudodiaptomus koreanus, Tortanus dextrilobatus, and Sinocalanus tenellus were highest in low salinity areas, followed by high salinity areas. However, the density of a few Pseudodiaptomus koreanus was somewhat higher in polyhaline zones which indicated that it is a highly diversified and retentive species. Tortanus dextrilobatus and Sinocalanus tenellus density was highest in oligohaline and mesohaline areas. Low density was also estimated in polyhaline zones (Figs. 4 and 5). The density of mesohaline species (Acartia ohtsukai and Acartia forticrusa) was usually high at the mesohaline zones. The density of Acartia ohtsukai was also found to be highest in the polyhaline and mesohaline areas, but the density of Acartia forticrusa was zero at polyhaline while few copepods were found in the oligohaline zone. They are widely distributed in the mesohaline zone (Figs. 4 and 5).
Fig. 4. Variation of copepods abundance (ind.m-3) at difference sampling stations in, (a) high tide/flood and (b) low tide/ebb of the Seomjin River estuary.
Fig. 5. Species level distribution pattern of copepods in both high tide and low tide of the Seomjin River estuary. (a, oligohaline of high/low tide; b, mesohaline of high/low tide and c, polyhaline of high/low tide.).
The densities of polyhaline species (Calanus sinicus, Paracalanus parvus s. l., Labidocera rotunda and Centropages dorsipinatus) were high in polyhaline areas while low in oligohaline areas during low tide (Fig. 4). During high tide, the density of Calanus sinicus, Paracalanus parvus s. l and Labidocera rotunda were highest in the polyhaline region, but the density of Centropages dorsispinatus was highest in the mesohaline region. This group of copepods were not observed in the oligohaline division but occasionally a few of them were found in this salinity range because of environmental factors (Figs. 4 and 5).
Overall copepod density varied between low and high tides with salinity; in high tide, the highest density was observed at mesohaline station 13 (5,113 ind.m-3) and the lowest was observed at oligohaline station 3 (56 ind.m-3) (Fig. 4a). On the other hand, at low tide the highest density of copepods was at polyhaline station 16 (2,457 ind.m-3) and the lowest was at oligohaline station 8 (85 ind.m-3) (Fig. 4b). The present result showed significant difference in density with different tides (p<0.05).
On the other hand, in high tide Pseudodiaptomus koreanus, Tortanus dextrilobatus, Paracalanus parvus s. l. showed significant difference in their densities between stations (p< 0.05), while Acartia ohtsukai was insignificant (p>0.05). In low tide, Pseudodiaptomus koreanus, Tortanus dextrilobatus, Acartia ohtsukai, Paracalanus parvus s. l. were gradually dominant along high salinity gradient, but only Pseudodiaptomus koreanus and Tortanus dextrilobatus showed significant difference in their densities among stations (p<0.05).
DISCUSSION
Estuarine copepods were well distributed and migrated from changing salinities in different stations. In the Seomjin River estuary, copepods display species-specific responses during high and low tides and copepods change in a particular habitat salinity zone caused by tide and salinity. Generally, estuarine copepods were dominant in their most favorable salinity zone, but they had to move to other salinity zones because of changing ecological processes. Despite of this fact, they are able to retain their density through horizontal or vertical migration strategies. Copepod populations make significant horizontal movements within the tidal region, where different densities are shown due to the influence of tides and salinity fluctuations. Higher densities and total abundances are found at high tide than at low tide (Robertson and Dixon 1988; Wang et al. 1995). When a river was swollen because of heavy rainfall, water flow was much faster than usual. At that time indigenous river-estuarine zooplankton will be swept out to sea despite of such maintenance mechanisms (Ueda et al. 2004). The present results have shown that population abundance was highest at high tidal zones than at low tidal zones because heavy rainfall broadened the adjacent flood area out from the river basin where the copepod population was randomly highly distributed, while the concentration of copepods was reduced due to a lack of suitable environmental conditions.
In the Seomjin River estuary, high and low tide migration, which occur four times, every six hours during a lunar day. They also include discharge, heavy rainfall during August, which affects the functioning of the reservoir ecosystem. Heavy rainfall flooded adjacent areas on the river bank, which caused salinity to dramatically change and tidal movements to activate the flood tidal ecology. Flood had a clear effect on zooplankton, both in concentration and composition (Godlewska1 et al. 2003). The positions of the mixing zone between the two water masses within the estuary will obviously change, not only with the ebb and flood flow of the tides, but also seasonally, depending on the volume of freshwater input. For example, Tortanus dextrilobatus was shown to be highest in the mesohaline zone at high tide, while usually being highest at oligohaline at low tide within the Seomjin River estuary. However, during ebb tides the density increased in the mesohaline zone and decreased in the oligohaline zone. The inability of coastal species to acclimatize to varying salinity of estuarine waters may be responsible for population declines. Like this, each copepod species has its own specific salinity zone, while particular physical environmental factors directly influence population transfer.
Ueda et al. (2004) reported that a gorge connected to the estuary of the river was important in maintaining the population of copepod species that move from the estuary to the bottom layer of the gorge. And another migration patterns have been identified in some copepods, which showed a trend of rapid downward migration from high to low tidal phases (Schlacher and Wooldridge 1995). In the mixing process, salinity underwent large variation and the influence of this change may not only vary from species to species, but also among different development stages (Lee and Peterson 2003), and the daily and seasonal salinity variations are important factors affecting copepod life cycle in estuarine conditions (Joan 1963). In this study, the response of copepods to instantaneous changes of salinity was to increase in numbers toward the sea and decrease in numbers toward the river. Some clearly adapted, while other were clearly influenced by salinity changes. Jeffries (1967) recognized the importance of salinity in a study on copepod spatial and temporal distributions. The distribution of species that inhabit estuaries was closely related not only to salinity but also to water temperature, food, and other factors (Uye et al. 1982). The survival of copepodites based on salinity change was clearly lower to that of adults (Guillermo et al. 1999). Zooplankton species in estuaries are often individually distributed along a salinity gradient (Miller 1983; Suh et al. 1991; Park et al. 2002). When a large volume of freshwater runoff meets the sea in areas such as estuaries, salinity can vary dramatically in both the horizontal and vertical planes. Horizontal salinity gradients are very well studied and often determine the horizontal distribution and density of organisms throughout an estuary (Kinne 1966; Ambler et al. 1985). The variable salinity in low and high tides clearly influenced population distribution and migration. Salinity induced stress, caused by any change in salinity from ambient (Kinne 1966) and tended to move in a particular area.
ACKNOWLEDGEMENTS
We would like to thank the biodiversity laboratory members at Chonnam National University for their assistance with sample collection.
Reference

2.Castel J and J Veiga. 1990. Distribution and retention of the copepods Eurytemora affinis hirundoides in a turbid estuary. Mar. Biol. 107:119-128.

3.Collins NR and R Williams. 1981. Zooplankton of the bristol channel and severn estuary, the distribution of four copepods in relation to salinity. Mar. Biol. 64:273-283.
4.Cronin LE, JC Daiber and EM Hulbert. 1962. Quantitative seasonal aspects of zooplankton in the Delaware River Estuary. Chesapeake Sci. 3:63-93.

5.Day JW, CA Hall, WM Kemp and AY Arancibia. 1989. Estuarine Ecology. John Wiley & Sons, New York, 559pp.
6.Godlewskal M, G Mazurkiewicz-Boro´n, A Pociecha, E Wilk- Wózniak and M Jelonek. 2003. Effects of flood on the functioning of the Dobczyce reservoir ecosystem. Hydrobiology 504:305- 313.

7.Guillermo C, G Raymond and P Marc. 1999. Influence of salinity on the distribution of Acartia tonsa (Copepoda, Calanoida). J. Exp. Mar. Biol. Ecol. 239:33-45.

8.Haettel L, C Osterberg, JR Curl and PK Park. 1969. Nutrient and plankton ecology of the Columbia River estuary. Ecology 50:962-978.

9.Hays GC, H Kennedy and BW Frost. 2001. Individual variability in diel vertical migration of a marine copepod: why some individuals remain at depth when others migrate. Limnol. Oceanogr. 46:2050-2054.

10.Head EJH, LR Harris and DC Abou. 1985. Effect of day length and food concentration on in situ diurnal feeding rhythms in Arctic copepods. Mar. Ecol. Prog. Ser. 24:281-288.

11.Hough AR and E Naylor. 1991. Field studies on retention of the planktonic copepod Eurytemora affinis in a mixed estuary. Mar. Ecol. Prog. Ser. 76:115-122.

12.Jeffries HP. 1967. Saturation of estuarine zooplankton by congeneric associates. pp.500-508. In Estuaries. (Lauff GH ed.).
13.Jerling HL and TH Wooldridge. 1991. Population dynamics and estimates of production for the calanoid copepod Pseudodiaptomus hessei in a warm temperate estuary. J. Estuar. Coast. Shelf Sci. 33:121-135.

14.Joan L. 1963. The salinity tolerance of some estuarine planktonic copepods. Limnol. Oceanogr. 8:440-449.

15.Kaartvedt S. 1993. Drifting and resident plankton. Bull. Mar. Sci. 53:154-159.
16.Kimmerer WJ, JR Burau and WA Bennett. 1998. Tidally oriented vertical migration and position maintenance of zooplankton in a temperate estuary. Limnol. Oceanogr. 43:1697-1709.

17.Kinne O. 1966. Physiological aspects of animal life in estuaries with special reference to salinity. Netherlands J. Sea Res. 3:222-244.

18.Laprise R and JJ Dodson. 1994. Environmental variability as a factor controlling spatial patterns in distribution and species diversity of zooplankton in the St. Lawrence Estuary. Mar. Ecol. Prog. Ser. 107:67-81.

19.Lee CE and CH Peterson. 2003. Effects of developmental acclimation on adult salinity tolerance in the freshwater-invading copepod Erytemora affinis. Physiol. Biochem. Zool. 76:296- 301.


20.Miller CB. 1983. The zooplankton of estuaries, pp.103-149. In Estuaries and Enclosed Seas (Ketchum BK ed.). Elsevier Scientific Publishing Company, Amsterdam.
21.Morgan CA, JR Cordell and CA Simenstad. 1997. Sink or swim? Copepod population maintenance in the Columbia River estuarine turbidity-maxima region. Mar. Biol. 129: 309-317.

22.Park C, PG Lee and SR Yang. 2002. Variation of zooplankton distribution in the Seomjin River estuary with respect to season and salinity gradient. The Sea, J. Korean Soc. Oceanogr. 7:51-59 (in Korean with English abstract).
23.Park EO, HL Suh and HY Soh. 2005. Seasonal variation in the abundance of the demersal copepod Pseudodiptomus sp. (Calanoida, Pseudodiaptomidae) in the Seomjin River Estuary, Southern Korea. Korean J. Environ. Biol. 23:367- 373.
24.Robertson AIP and PA Dixon. 1988. Zooplankton dynamics in mangrove and other near shore habitats in tropical Australia. Mar. Ecol. Prog. Ser. 43:139-150.

25.Schlacher TA and TH Wooldridge. 1995. Small-scale distribution and variability of demersal zooplankton in shallow, temperate estuary: tidal and depth effects on species-specific heterogeneity. Cah. Biol. Mar. 36:211-227.
26.Suh H-L, HY Soh and SS Cha. 1991. Salinity and distribution of zooplankton in the estuarine system of Mankyung River and Dongjin River. J. Oceanol. Soc. Korea 26:181-192 (in Korean with English abstract).
27.Ueda H, M Terao, M Tanaka, M Hibino and MS Islam. 2004. How can river estuarine planktonic copepods survive river floods?. Ecol. Res. 19:625- 632.

28.Uye S, Y Iwai and S Kasahara. 1982. Reproductive biology of Pseudodiaptomus marinus (Cpepoda: Calanoida) in the inland sea of Japan. Bull. Plank. Soc. Jap. 29:25-35.
29.Wang Z, E Thiebaut and JC Dauvin. 1995. Short-term variations of zooplankton in a megatidal estuary (Seine, eastern English Channel). Proc. the 20th Symp. Union des Ocenographes de.
30.Wooldridge T and T Erasmus. 1980. Utilization of tidal currents by estuarine zooplankton. J. Estuar. Coast. Shelf Sci. 11:107- 114.

Vol. 40 No. 4 (2022.12)
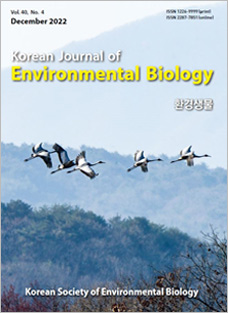
Frequency quarterly
Doi Prefix 10.11626/KJEB.
Year of Launching 1983
Publisher Korean Society of Environmental Biology




Online Submission
submission.koseb.org
KOSEB
Korean Society of Environmental Biology
Contact info
Any inquiries concerning Journal (all manuscripts,
reviews, and notes) should be addressed to the managing
editor of the Korean Society of Environmental Biology.
Yongeun Kim,
Korea University, Seoul 02841, Korea.
E-mail: kyezzz@korea.ac.kr /
Tel: +82-2-3290-3496 / +82-10-9516-1611