Journal Search Engine
ISSN : 2287-7851(Online)
Morphological and Molecular Analyses of Anabaena variabilis and Trichormus variabilis (Cyanobacteria) from Korea
Abstract
- 08-Morph-(환생)컬러.152522.pdf318.4KB
INTRODUCTION
Phylogenetic researches of cyanobacteria have established that phylogenetic relationships sometimes conflict with the morphological classification (Rajaniemi et al. 2005). The comparison of morphological and phylogenetical data is hindered by the lack of cultures of several cyanobacterial morphospecies and inadequate morphological properties of sequenced strains. Furthermore, it is not common for the names of cultured strains to be corrected or updated based on subsequent studies, or misidentifications of cultured strains to be corrected in collection list and databases. Phylogenetic analyses derived from molecular analyses of misidentified cultured strains thus may not reflect new results from morphological studies. Anabaena and Trichormus belong to subsection IV. I based on a bacteriological classification (Rippka et al. 2001), and the order Nostocales and family Nostocaceae according to traditional classification (Komárek and Anagnostidis 1989), with Anabaena variabilis Kützing, Anabaena flos-aquae(Lyngb.) De Brébisson and Trichormus variabilis (Kützingex Bornet et Flahault) Komárek et Anagnostidis as the type species, respectively (AlgaeBase 2007). The genus Anabaena is characterized by unbranched trichomes that possess intercalary heterocysts (Whitton 2002), and includes 34 species (NCBI 2011).To examine morphological variations among Korea Anabaena and Trichormus strains, we collected many samples from different localities throughout Korea, obtaining more than ten strains. This study focused on the combined molecular and morphological relationships of the two genera Trichormus and Anabaena. Five Trichormus and 3 Anabaena strains isolated from Korea and 3 A. flos-aquae strains from UTEX(Texas, USA) were identified based on their morphology and molecular analyses.
MATERIALS AND METHODS
1. Isolation and culture of Trichormus and Anabaena strains
Single trichomes of cyanobacteria were isolated from six surface-water samples from rivers and reservoirs in Korea(Table 1). The isolated strains were identified as Trichormus and Anabaena using a light microscope. Meanwhile, three A. flos-aquae strains were obtained from UTEX Culture Collection of Algae. The isolation and culturing of these strains were carried out using standard procedures. The purified unialgal filaments were plated on 1.2% (w/v) agarose (QAAgarose TM, Qbiogene Inc., OH, USA) plates prepared in a BG-11 medium (Rippka 1988). All the strains were derived from a single trichome using a modified micromanipulator method (Oh and Rhee 1990). The purified filaments growing on the plate were then inoculated into a liquid BG-11 medium and cultured at 26±1°C at a photosynthetic photon flux density of 100 μmol photons m-2 s-1 with cool white light fluorescent lamps (HYG-FPL36W, Heat Engineering Co.,Korea) and continuous illumination.
2. Morphological analysis
Strains were indentified according to Komárek and Anagnostidis(1989). Morphological and morphometrical studies were measured based on 30 individuals per strain using a light microscope (Microphoto-FXA, Nikon Co., Japan). Micrographs were taken with a Nikon CCD camera system (Digital Sight DS-5Mc, Nikon Co., Japan). The parameters of isolated strains examined in this study were length and width of vegetative cells, heterocysts, and akinetes and shape of apices under the microscope using 400 times objective lenses.
3. DNA Extraction, PCR amplification, and
The cells were harvested in the late exponential phase by centrifugation for 10 min at 15,000 xg (4°C) and stored at -20°C. The DNA was extracted using an YGB100 Genomic DNA extraction kit (Real Biotech Co., Taiwan). The 16SrRNA gene was then amplified using the universal primer 27F (5′ AGA GTT TGA TCM TGG CTC AG) and 1522R (5′ AAG GAG GTG WTC CAR CC) (Cho and Giovannoni 2003), while the cpcBA-IGS was amplified using CPC1F (5′ GGC KGC YTG YYT RCG YGA CAT GGA 3′) and CPC1R (5′ AAR CGN CCT TGR GWA TCD GC 3′) (Kim et al. 2006). The amplification was carried out in 50 μL of a 1x nTaq buffer, 0.2 mM of each dNTP, 10 pmol of each primer, 2.5 U of nTaq polymerase (Biostream Ltd., Korea), and 1 μL (ca. 20 ng) of the isolated DNA. The amplification parameters for the 16S rRNA gene were 94°C for 4 min, followed by 30 cycles of 94°C for 1 min, 55°C for 1 min, and 72°C for 1.5 min, plus an extension at 72°C for 7 min. For the cpcBA-IGS, the amplification parameters were 94°C for 4min, followed by 30 cycles of 94°C for 30 sec, 55°C for 30sec, and 72°C for 45 sec, plus an extension at 72°C for 7 min. The presence of a PCR product was confirmed on a 0.8%LF agarose gel(VentechBio Ltd., Korea) run at 100 V with 2 μL of the PCR product and 100 ng of a 1 Kb ladder molecular size marker (Fermentor Ltd., Korea). The PCR products were purified using a Hiyield Gel/PCR DNA fragment Extraction Kit (Real Biotech, Taiwan), then cloned with a p-Drive T-cloning vector (Qiagene Co., Japan). The plasmids were transferred to Escherichia coli DH-5α(Real Biotech Co., Taiwan) and isolated using a Hiyield plasmid mini kit (Real Biotech Co., Taiwan). The plasmids were then sequenced (Cosmogen Ltd., Korea) bidirectionally with SP6, T7, and internal sequencing primers 1100R (5′ GGG TTG CGC TCG TTG-3′) for the 16S rRNA gene sequences, and SP6 and T7 for the cpcBA-IGS sequences. The GenBank accession numbers for the 16S rDNA and cpcBA-IGS are as follows: DQ234812-DQ234833.
Table 1. Cyanobacterial strains used for morphological and phylogenetic analyses in this study
4. Sequence alignment and phylogenetic analyses
CLUSTAL-X was used to produce working alignments of the 16S rRNA gene and cpcBA-IGS sequences for the target strains. The final alignments were obtained by manual refinement, and ambiguous bases and hypervariable regions removed using PHYDIT 3.0 (Chun and Goodfellow 1995). As the highly variable intergenic spacer (IGS) region between the cpcB and cpcA genes did not allow a reliable alignment, these regions were excluded from the analysis. The alignments used in the phylogenetic analyses consisted of 1,302bp (16S rRNA gene sequences) and 297 bp (cpcBA-IGS sequences). Phylogenetic trees were constructed using the neighbor-joining (NJ) and maximum-parsimony (MP) method from the TREECON software package (Van de Peer and DeWachter 1994). The statistical significance of the branches was estimated by a bootstrap analysis of the tree programs, involving the generation of 1,000 trees. The distances for the NJ tree were estimated using the algorithm of Kimura(Kimura 1980).
RESULTS
1. Morphological analysis
The trichomes and cells in the preserved samples exhibited typical morphological features of species of Trichormus and Anabaena, respectively (Fig. 1). According to traditional morphological criteria, among the examined 11 strains, 5 were benthic and identified as Trichormus variabilis, while 6 were planktonic and identified as Anabaena variabilis and A. flos-aquae. The trichomes of the isolated Trichormus strains were entangled and sank to the bottom of the culture flasks. Under the light microscope, the trichomes were observed as unsheathed, generally immobile, straight, and sometimes bent. The trichomes of the isolated Anabaena strains were solitary. Under the light microscope, the trichomes of the A. variabilis were single and straight formed. But the trichomes of the A. flos-aquae were coiled forming a solitary irregular spiral fashion. The morphological characteristics of the strains are summarized in Table 2. The shapes of the terminal cells were rounded in the case of 8 strains, conical in 2 strains, and tapered in 1 strain. The cells of T. variabilis were 4.1 to 5.8μm long and 3.5 to 5.6 wide, plus the cell length to width ratio varied from 0.85 to 1.19. The trichomes divided transversely and intercellularly, and were composed of cylindrical cells. The cells of A. variabilis were 5.5 to 6.0 μm long and 2.5 to 3.8 μm wide, plus the cell length to width ratio varied from 1.45 to 2.40. The trichomes divided transversely and intercellularly, and were composed of cylindrical cells. The apical cells were generally rounded or long-pointed after a trichome breakage and had no calyptra. Meanwhile, the cells of A. flos-aquae were 5.8 to 6.2 μm long and 4.1 to 4.2μm wide, plus the cell length to width ratio varied from 1.38to 1.48.
The Trichormus and Anabaena strains differed significantly in the length to width ratio of the vegetative cell and akinetes, where the A. variabilis strains showed wider vegetative cell and akinetes in comparison to the T. variabilis strains, indicating that the length of the heterocyst was a taxonomic character. Plus, the morphology of the end cells of the
trichomes in the Trichormus strains was rounded, conical, and tapered, while that in the Anabaena strains was rounded.
Fig. 1. Light microphotoimages of 5 Trichormus variabilis strains (A), 3 Anabaena variabilis strains (B), and 3 Anabaena flos-aquae strains (C). Magnification=400, Scale Bars=20 μm(A: akinete, H: heterocyst).
2. Sequence of the 16S rRNA and cpcBA-IGS
Near-full-length 16S rRNA gene sequences (1,444 bp) were determined for the 3 A. variabilis strains and 5 T. variabilis strains isolated from Korea and the 3 A. flos-aquae strains from UTEX. GenBank accession numbers are given in Table 1. The overall similarity of the 3 A. variabilis strains (KCTCAG10059, KCTC AG10064, and KCTC AG10273) was >
99.9%, and among these 3 strains, the similarity with A. variabilis ATCC 29413 was 99% (Table 3). Meanwhile, the 5T. variabilis strains (KCTC AG10008, KCTC AG10026, KCTC AG10178, KCTC AG10180, and KCTC AG10269) shared high similarity values of at least 99.9%, and the similarity between 5 strains and T. variabilis GREIFSWALD was 99% (Table 3). The 3 A. flos-aquae strains (UTEX LB2557, LB2391, and LB2383) shared similarity values of >99.7%, and the similarity with Nostoc entophytum IAM M-267 and N. linckia was 99% and 98%, respectively (Table 3). The less conserved IGS between cpcB and cpcA, and corresponding flanking regions (henceforth referred to as cpcBA-IGS) were also sequenced for the strains examined in this study. The region covered the end of the cpcB gene (259 bp), an intergenic spacer (IGS, 90~104 bp), and the end of the cpcA gene (33 bp). The overall similarity of the 3 A. variabilis strains (KCTC AG10059, KCTC AG10064, and KCTC AG10273) was up to >99.5%, and among these 3 strains, the similarity with Nostoc sp. PCC 7120 was 99% (Table 3). The 5 T. variabilis strains (KCTC AG10008, KCTC AG10026, KCTC AG10178, KCTC AG10180, and KCTC AG10269) shared high similarity values up to at least 99.5% and the similarity between 5 strains and A. compacta 1403/24 was 93~94% (Table 3). The 3 A. flos-aquae strains (UTEX LB2557, LB2391, and LB2383) shared similarity values of >99.0%, and the similarity with Aphanizomenon flos-aquae CCAP 1401/1 was 97% (Table 3).
Table 2. Selected morphological characteristics of cyanobacterial strains studied
Table 3. Sequence identities of cyanobacterial strains in this study based on nucleotide-nucleotide BLAST (blastn) of the National Center for Biotechnology Information (NCBI)
Fig. 2. Phylogenetic tree generated via the neighbor-joining method showing the phylogenetic relationships of the Anabaena and Trichormus strains based on the analysis of ca. 1300 bases of aligned 16S rRNA gene sequences. Sequences obtained during the present study are indicated in bold. Numbers near nodes indicate bootstrap values over or equal to 50% for NJ and MP analyses. Sequences from GenBank are indicated by accession numbers. Outgroup was Escherichia coli K-12 (AE000460). The scale bar represents 0.02 changes per nucleotide.
3. Genetic relationships of the studied strains
Since the neighbor-joining (NJ) and maximum-parsimony (MP) trees were similar, only the NJ tree is presented to show the phylogenetic relationship of the cyanobacterial strains for each gene (Figs. 2 and 3). The GenBank accession numbers are shown Table 1. A phylogenetic 16S rRNA gene trees were reconstructed from an alignment of 43 sequences, including 6 Anabaena and 5 Trichormus isolates, representing the major lineage of cyanobacteria with Escherichia coli as the outgroup (Fig. 2). The cyanobacteria studied here were divided into three main groups, where the first consisted of filamentous cyanobacteria, Anabaena and Nodularia, capable of forming heterocysts and akinetes, the second contained non-heterocystous, filamentous Oscillatoria, Lyngbya, and Arthrospira strains, and the third contained unicellular Microcystis, Synechocystis, and Synechococcus strains. The 5 T. variabilis strains (KCTC AG10008, KCTC AG10026, KCTC AG10178, KCTC AG10180, and KCTC AG10269) clustered with T. variabilis GREIFSWALD. These six sequences shared greater than 99.8% sequence similarity and were supported by 99% bootstrap resamplings in both NJ and MP analysis. Trichormus strains, which were separated morphologically from Anabaena strains, were divided into 3 well-separated clusters and were not monophyletic. The 3A. variabilis strains (KCTC AG10059, KCTC AG10064, and KCTC AG10273), sequenced in this study, clustered with A. variabilis ATCC 29413, A. variabilis NIES 23, and A. flosaquae UTCC 64 and supported by 100% bootstrap resamplings in both analysis. The 3 A. flos-aquae strains (UTEXLB2557, LB2391, and LB2383), which classified with A. flos-aquae by morphological analysis, clustered with N. entophytum IAM M-267 with high bootstrap support and might actually belong to the genus Nostoc rather than to Trichormus or Anabaena. A. flos-aquae strains (1tu35s12, UTCC 64, and NIES 73) were dispersed in Anabaena subclusters. The 37 sequences of cpcBA-IGS, including 6 Anabaena and 5 Trichormus isolates, were used in the both (NJ and MP) phylogenetic analysis (Fig. 3). To compare the congruence between the 16S rRNA gene and cpcBA-IGS sequences based on the phylogenies of Nostocaceae, 17 strains with both 16S rRNA and cpcBA-IGS sequences were selected from the NCBI database. Unfortunately, the cpcBA-IGS sequence of T. variabilis was not found in the NCBI database. A phylogenetic tree was constructed based on an unambiguous alignment of 297 bp (Fig. 3). Due to the highly variable nature of the IGS sequence and the resulting alignment difficulties, these 297 bp originated almost exclusively from within the coding regions of cpcB and cpcA flanking the IGS. The division of the more distantly related groups was in agreement with the previously determined 16S rRNA gene-based phylogeny. The Synechococcus strains were the deepest branching group in the 16S rRNA gene tree. Similarly, the Arthrospira strains associated with Lyngbya aestuarii PCC 7419 in both the cpcBA-IGS and 16S rRNA gene-based tree. The two sequences were supported by 100% bootstrap values in both NJ and MP analysis. Although Oscillatoria sancta PCC 7515 was associated with L. aestuarii PCC 7419 in the 16S rRNA gene tree, O. sancta PCC 7515 was interestingly associated with M. aeruginosa NIES 98 according to the cpcBA-IGS trees. The A. flos-aquae strains (UTEX LB2557, LB2391, and LB2383) were associated with the A. variabilis strains in the 16S rRNA gene tree. However, according to the cpcBA-IGS sequence tree, the A. flos-aquae strains were associated with the T. variabilis strains.
Fig. 3. Phylogenetic tree based on cpcBA-IGS sequences (297 bp), showing clustering of examined Anabaena and Trichormus strains. Sequences obtained during the present study are indicated in bold. Numbers near nodes indicate bootstrap values over or equal to 50% for NJ and MP analyses. Sequences from GenBank are indicated by accession numbers. Outgroup was chloroplast Cyanidium caldarium (S77125). The scale bar represents 0.05 changes per nucleotide.
DISCUSSION
Analyses of the 16S rRNA gene and cpcBA-IGS sequences of the Anabaena and Trichormus strains showed a high similarity between the A. variabilis and T. variabilis strains and did not confirm the taxonomic validity of these two genera. Phylogenetic analyses based on 16S rDNA sequences have already been widely used. However, the 16S rRNA gene is often ineffective for resolving bacterial strains, due to its slow rate of evolution. In addition to analyzing the 16S rDNA sequences (Neilan et al. 1997; Otsuka et al. 1998; Honda et al. 1999), the genes encoding the major light-harvesting accessory pigment proteins, particularly the phycocyanin operon(cpc), including the intergenic spacer (IGS) between cpcB and cpcA and the corresponding flanking regions (cpcBAIGS), have been targeted for phylogenetic studies of cyanobacteria (Barker et al. 1999). Phycocyanins are specific accessory pigments in Cyanophyta, Rhodophyta, Glaucophyta, and Cryptophyta. In a previous report (Teneva et al. 2005), the length of the IGS separating cpcB and cpcA was found to exhibit a strong relationship with the phylogenetic groups. However, there were generally lower in the cpcBA-IGS tree than in the 16S rRNA gene tree, due to a smaller number of cpcBA-IGS data bases. Moreover, to define and delimit the genus Anabaena and Trichormus, this study also included other cyanobacteria, and a further goal was to identify specific molecular markers to fingerprint different strains of Anabaena and Trichormus and enable the development of tools for strain-specific identification. The phylogenetic analysis of the 16S rDNA sequences further reinforced the polyphyletic nature of this taxon. The difference between the molecular and morphological results may have reflected the existence of species-variant populations or ecotypes adapted to different environmental conditions. Recently, Baker et al. (2001, 2002) employed a PCR amplification method for the analysis of cpcBA from environmental samples using a primer set previously designed by Neilan et al. (1997) and found a limited cyanobacterial diversity. Although the primer set was originally designed with 6 cpcBA sequences to study the genetic diversity of several pure culture strains, cpcBA sequence information from various other cyanobacteria has also been deposited in public databases for potential enhanced primer design. Furthermore, since the IGS length of each group of cyanobacteria is different, the cpcBA gene amplification protocol could be directly coupled to length polymorphism analysis techniques, such as TRFLP, to facilitate rapid monitoring of the cyanobacterial community. In conclusion, Trichormus and Anabaena strains, which were isolated from Korean waters, were separated by morphological and phylogenetic analyses. However, the difference remained between morphology and phylogeny of Trichormus and Anabaena strains. The phylogenetic relationship of the studied strains did not follow the current taxonomic classification and therefore a revision of the taxonomy of these anabaenoid strains is needed.
ACKNOWLEDGMENT
This study was supported by grants from the Carbon Dioxide Reduction and Sequestration Research Center (CDRS), a 21st Century Frontier Program and the Advanced Biomass R&D Center (ABC), a Global Frontier Program funded by the Korean Ministry of Education, Science & Technology(MEST).
Reference






















Vol. 40 No. 4 (2022.12)
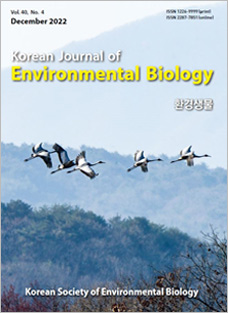
Frequency quarterly
Doi Prefix 10.11626/KJEB.
Year of Launching 1983
Publisher Korean Society of Environmental Biology




Online Submission
submission.koseb.org
KOSEB
Korean Society of Environmental Biology
Contact info
Any inquiries concerning Journal (all manuscripts,
reviews, and notes) should be addressed to the managing
editor of the Korean Society of Environmental Biology.
Yongeun Kim,
Korea University, Seoul 02841, Korea.
E-mail: kyezzz@korea.ac.kr /
Tel: +82-2-3290-3496 / +82-10-9516-1611