Journal Search Engine
ISSN : 2287-7851(Online)
Fluctuation Rates of Phytoplankton Assemblages by Passage through Power Plant Cooling System
Abstract
- 23201130.pdf527.1KB
INTRODUCTION
The seawater is generally used as a cooling agent. This is common practice because power plants need a great deal of cooling agents in its daily operation. Nuclear power plants need cooling waters more than thermal power plants because of the lower thermal efficient. Consequently, these cooling waters-called thermal discharges or thermal effluents-enter waters adjacent to these power plants. These thermal discharges have an influence on marine ecosystems and often limit the distribution of marine organisms including phytoplankton at discharge areas (Langford 1990; Suresh et al. 1995).
Principal factors affecting organisms, in thermal discharges of power plants are (1) thermal shock due to abrupt changes in water temperature (2) chlorination of the discharge water, and (3) elevated temperatures caused by cooling water discharge. Chlorine, used to control slime build-up in power plant cooling systems, inhibits the growth of phytoplankton assemblages (e.g. Morgan and Stross 1969; Hamilton et al. 1970; Brook and Baker 1972; Carpenter et al. 1972). Among the several groups of marine organisms that can be entrained, phytoplankton have special significance because they contribute a basic link the aquatic food chain. The entrainment effects on phytoplankton are variable and depend on the location and operating conditions of power plant (Warinner and Brehmer 1966; Hamilton et al. 1970; Brook and Baker 1972; Carpenter et al. 1972; Fox and Moyer 1973, 1975). Most attention has been focused on the effects of temperature increases and chlorination; however, chlorination is normally associated with estuarine and marine environments which are typically populated with fouling communities. The concentration of chlorophyll a in a standing crop was measured to estimate effects on the phytoplankton. The result of this measurement was a photosynthesis index rate of 14C. Carbon assimilation, chlorophyll a and various physiological indices were used to measure the response of phytoplankton entrainment in once-through cooling waters at the power plant (Dunstall 1985). Some studies were conducted on phytoplankton community structure and the influence of thermal discharges on phytoplankton in the adjacent waters of power plants in Korea (Yi and Chin 1987; Yeo and Shim 1992; Lee and Lee 1997; Kang and Choi 2001, 2002; Kang et al. 2003; Kang 2008). These studies performed around power plants were mainly focused on ecological aspects of phytoplankton ecology, i.e. temporal and spatial variation of species composition, standing crops and dominant species. The entrainment effects on phytoplankton passing through the cooling systems of power plants have yet to be fully understood (Yeo and Kim 1998; Choi et al. 2004).
Wolsong nuclear power plant (NPP) is located in the coastal area of Kyeongju, East Sea (35o 43′35N, 129o 30′35′′). The present unit began operation in 1986. Electrical generating capacity of the plant is 260 MW. Circulation pumps move water through the condenser system at the rate of about 40~50m3 sec-1. Chlorine was injected continuously in the mouth of intake at concentrations of about 0.33 mg L-1. As part of normal plant operations, there was a low concentration of usually less than 0.1 mg L-1 chlorine at the front of outlet.
In this study, we tried to analyze the standing crops, chlorophyll a concentrations, and carbon assimilation number of phytoplankton before and after PTCS of Wolsong NPP-gain a more information of entrainment effect on phytoplankton communities.
MATERIALS AND METHODS
Thermal discharges from Wolsong NPP were used for the field examination and laboratory experiment of possible thermal and chlorination effects. Samples of intake and outlet water were taken from July 2006 to June 2008. Standing crops, chlorophyll a concentrations, and carbon assimilation number of phytoplankton were made. Residence time of cooling waters between the two sampling points (A, B) is approximately 15 minutes, but in reality depends on the rate of power plant operation. Sampling stations for the intake and outlet are shown in Fig. 1. The sea surface temperature (SST), salinity, and pH at intake and outlet were measured by CTD meter (IDRONAUT 316).
Fig. 1. Map showing the sampling station at the Wolsong NPP (A: Intake, B: Outlet).
Standing crops, carbon assimilation numbers, and chlorophyll a concentrations were measured before and after PTCS. Phytoplankton samples were counted using a microscope with 150× magnification and filtered through a glass filter fiber (GF/F, Whatman). The procedure from Parsons et al. (1984) was used to determine the chlorophyll a concentrations. The phytoplankton carbon assimilation number was measured by the 14C-technique based on Parson et al. (1984). The results were expressed as mean counts of duplicate samples per two hours of incubation. Water samples for measuring chlorophyll a concentrations were size-fractionated with separate filtration: microplankton (>20 μm), nanoplankton (5~20 μm) and picoplankton (<5 μm). Filtered seawater was kept deep frozen and analyzed at the laboratory on Turner 10-AU fluorometer. In field 14C experiments, phytoplankton samples were incubated in constant artificial light and temperature-controlled water baths in all of the studies. The heating-cooling apparatus employed was a spiral glass tubing 3 cm in diameter and about 30 cm in length. It was submerged in a thermostatic water bath in situ.
The fluctuation rates (FR) of standing crops, chlorophyll a concentrations, and carbon assimilation number of samples were compared in the intake and outlet water of Wolsong NPP. The FR of phytoplankton by PTCS was calculated by the following equation:
The value of IntakeSC, Chl, CA and OutletSC, Chl, CA represent the standing crops (SC), chlorophyll a concentrations (Chl) and carbon assimilation number (CA) of phytoplankton at the intake and outlet, respectively.
RESULTS
1. Distribution of temperature, salinity, and pH
The SST varied from 11.4~24.8oC and 15.4~29.0oC in intake and outlet during the survey periods, respectively (Fig. 2A). So the differences of SST between intake and outlet varied from 1.4 (November 2006)~6.2 (September 2006)oC with the operation conditions. The SST varied 11.4~13.5 (mean 12.7)oC, 12.4~19.7 (mean 16.0)oC, 16.9~24.8 (mean 21.1)oC and 13.9~19.7 (mean 16.6)oC in winter, spring, summer and autumn, respectively. The salinity of intake and outlet varied from 31.4~34.4 psu and 30.7~34.2 psu, respectively (Fig. 2B). The variations of pH in the intake and outlet varied from 7.9~8.3 and 8.0~8.3, respectively (Fig. 2C). So there was little difference in salinity and pH between the intake and outlet.
Fig. 2. Changes in temperature (A), salinity (B) and pH (C) at intake and outlet of Wolsong NPP from July 2006 to June 2008.
2. FR of the standing crops by PTCS
The standing crops of phytoplankton were compared in water samples at intake and outlet of Wolsong NPP. The total standing crops of phytoplankton ranged from 3.9×105 cells L-1 to 5.2×106 (mean 1.0×106) cells L-1 at intake and from 2.5×106 cells L-1 to 3.4×106 (mean 7.3×105) cells L-1 at outlet (Table 1). The total standing crops of phytoplankton were reduced by PTCS during the survey periods and the amount of mean decrease was 2.7×105 cells L-1. The FR of standing phytoplankton crops was -16.0~59.3 (mean 27.0)% (Fig. 3A). They were markedly reduced in July (45.3%) 2006 and April (59.3%) 2007. The total standing crops of phytoplankton at outlet, however, were higher than those of them at intake in May, June 2007 and April 2008 (Table 1). The standing crops of microplankton (>20 μm) ranged from 9.8×104 cells L-1 to 4.9×106 (mean 6.7×105) cells L-1 at intake and from 5.6×104 cells L-1 to 2.7 ×106 (mean 4.5×105 cells L-1 at outlet (Table 1). The amount of mean decrease was 2.3×105 cells L-1. So the FR of total standing crops of microplankton was -21.4~61.7 (mean 34.1%) (Fig. 3A). They were markedly reduced in July 2006 (50.6%) and April 2007 (61.7%). The standing crops of microplankton at outlet, however, were higher than those of them at intake in May, June 2007 and June 2008 (Fig. 3A).
Table 1. The standing crops of phytoplankton at intake and outlet of Wolsong NPP from July 2006 to June 2008 (Units: ×103 cells L-1)
Fig. 3. Changes in FR of microplankton, nanoplankton, total phytoplankton, diatoms and dinoflagellates by PTCS of Wolsong NPP from July 2006 to June 2008.
Among microplankton, the amount of mean decrease of diatoms was 2.1×105 cells L-1 (Table 1). So the FR of diatoms was -17.5~61.9 (mean 33.9%) (Fig. 3B). They were markedly reduced in July 2006 (50.8%) and March (47.7%) and April (61.9%) 2007. In November 2006, January, May, and June 2007, and June 2008, however, the standing crops of diatoms at outlet were higher than those of them at intake. The amount of mean decrease of dinoflagellates was 1.4×104 cells L-1 (Table 1). So the mean FR of dinoflagellates was 29.7%. They were markedly reduced in September (57.9%), November (57.9%) 2006, March 2007 (47.7%) and February 2008 (61.9%) (Fig. 3B). In March and June 2007 and April and June 2008, however, the standing crops of dinoflagellates at outlet were higher than those of them at intake. In addition, the mean FR of other groups (Cyanophyta, Chrysophyta, Euglena, etc.) was 17.6%.
On the other hand, the standing crops of nanoplankton (< 20 μm) ranged from 5.9×104 cells L-1 to 8.4×105 (mean 3.3×105) cells L-1 and from 4.2×104 cells L-1 to 6.8×105 (mean 2.9×105) cells L-1 at intake and outlet, respectively (Table 1). The amount of mean decrease was 4.1×104 cells L-1. So the FR of nanoplankton was -24.2~49.9 (mean 12.4)% (Fig. 3A). There were markedly reduced in July (33.5%) and November (35.8%) 2006 and June 2008 (49.9%). The standing crops of nanoplankton at outlet, however, were higher than those of them at intake in August 2006, January and June 2007, and April 2008.
Accordingly, the mean FR of standing crops of microplankton, nanoplankton, diatoms, dinoflagellates and other groups during the survey periods was 34.1%, 12.4%, 33.9%, 29.7% and 17.6%, respectively (Fig. 3A, B). The seasonal changes in FR of microplankton were 1.7~32.3%, and the highest and lowest value were found in spring and winter, respectively. But the seasonal changes in mean FR of nanoplankton were 10.3~15.7%, so a little was differences in seasons (Fig. 4). The seasonal changes in mean FR of diatom were 4.0~30.8%, and the highest and lowest value were found in spring and winter, respectively. The seasonal changes in mean FR of dinoflagellate were -4.2~44.0%, and the highest value was found in summer (Fig. 4). The diatoms and dinoflagellates appeared as a dominant groups during this survey, the diatoms significantly controlled the overall variations of standing crops of phytoplankton while passing through the cooling systems.
Fig. 4. Seasonal changes in FR of microplankton, nanoplankton, diatoms and dinoflagellates by PTCS of Wolsong NPP from July 2006 to June 2008.
These results showed that the FR of microplankton was higher than that of nanoplankton and the FR of diatoms was higher than that of dinoflagellates. Our results, therefore, indicated that the entrainment effects on microplankton and diatoms were higher than those of nanoplankton and dinoflagellates. In addition, phytoplankton decrement by PTCS was high at time when the standing crops of phytoplankton were high. But the decrement of phytoplankton was generally low at time when the standing crops of phytoplankton were low.
3. FR of the major species by PTCS
The standing crops of Chaetoceros debilis ranged from 6.4×102 cells L-1 to 3.8×105 (mean 7.5×104) cells L-1 at intake and from 4.9×102 cells L-1 to 4.5×105 (mean 6.1×104) cells L-1 at outlet. The standing crops of C. debilis were slightly reduced and the amount of mean decrease was 1.3×104 cells L-1. So the FR of standing crops of C. debilis was -70.0~84.9 (mean 17.8)% (Fig. 5). They were markedly reduced in March (72.7%) and November (84.9%) 2007 and February (72.9%) and March (75.2%) 2008. The standing crops of C. debilis at outlet, however, were higher than those at intake in May and June 2007. The standing crops of C. socialis ranged from 0 to 4.4×106 (mean 2.8×105) cells L-1 at the intake and from 0 to 1.6×106 (mean 1.0×105) cells L-1 at outlet. The standing crops of C. socialis were markedly reduced and the amount of mean decrease was 1.7×105 cells L-1. So the FR of standing crops of C. socialis was 8.7~100.0 (mean 62.8%) (Fig. 5). The standing crops of Leptocylindrus danicus ranged from 0 to 5.2×104 (mean 1.3×104) cells L-1 at intake and from 0 to 7.0×104 (mean 1.1×104) cells L-1 at outlet. The standing crops of L. danicus were slightly reduced and the amount of mean decrease was 1.8×103 cells L-1. So the mean FR of standing crops of L. danicus was 14.2% (Fig. 5). The standing crops of Pseudo-nitzschia seriata ranged from 0 to 5.2×104 (mean 1.0×104) cells L-1 at intake and from 0 to 7.6×104 (mean 1.1×104) cells L-1 at outlet. The standing crops of P. seriata were slightly reduced and the mean decrease was 1.8×103 cells L-1. So the mean FR of standing crops of P. seriata was 26.9% (Fig. 5). The standing crops of Skeletonema costatum-like species ranged from 0 to 1.5×104 (mean 4.9×103) cells L-1 at intake and from 0 to 1.6×104 (mean 4.2×103) cells L-1 at outlet. So the FR of S. costatum-like species was -142.7~100.0 (mean 14.0%) (Fig. 5). The standing crops of Thalassiosira decipiens ranged from 5.2×103 cells L-1 to 3.0×106 (mean 5.6×104) cells L-1 at intake and from 4.4×103 cells L-1 to 1.8×105 (mean 5.1×104) cells L-1 at outlet. The standing crops of T. decipiens were reduced the amount of mean decrease was 5.3×103 cells L-1. So the mean FR of standing crops of T. decipiens was 9.5% (Fig. 5). The standing crops of Prorocentrum sp. ranged from 5.4×102 to 4.2×104 (mean 1.2×104) cells L-1 at intake and from 5.4×102 cells L-1 to 2.3×104 (mean 8.5×103) cells L-1 at outlet. The mean decrease of Prorocentrum sp. was 3,983 cells L-1. So the mean FR of standing crops of Prorocentrum sp. was 31.8% (Fig. 5).
Fig. 5. Changes in FR of major species by PTCS of Wolsong NPP from July 2006 to June 2008.
4. FR of chlorophyll a concentrations by PTCS
The chlorophyll a concentrations of phytoplankton were compared in the samples of the intake and outlet of Wolsong NPP. The total chlorophyll a concentrations ranged from 0.40 μg L-1 to 6.47 (mean 1.79) μg L-1 at intake and from 0.30 μg L-1 to 2.54 (mean 0.82) μg L-1 at outlet. The amount of mean decrease of chlorophyll a concentrations was 0.97 μg L-1. So the FR of total chlorophyll a concentrations was 25.0~74.0 (mean 54.4)%. There were markedly reduced in July (72.5%) and August (74.0%) 2006 and April 2007 (63.2%) (Fig. 7).
Fig. 7. The yearly (A) and seasonal (B) changes in FR of chlorophyll a concentrations by PTCS of Wolsong NPP from July 2006 to June 2008.
The chlorophyll a concentrations of microplankton ranged from 0.06 μg L-1 to 5.50 (mean 1.12) μg L-1 at intake and from 0.04 μg L-1 to 1.56 (mean 0.46) μg L-1 at the outlet (Fig. 6A). The amount of mean decrease in chlorophyll a concentrations was 0.66 μg L-1. So the FR of chlorophyll a concentrations of microplankton was 7.7~81.5 (mean 58.9)% (Fig. 7A). They were markedly reduced in July (71.6%), August (81.5%), and December (68.0%) 2006 and April 2007 (68.1%). But, there was little difference in FR between intake and outlet in March (9.5%) and June (7.7%) 2007.
Fig. 6. Changes in chlorophyll a concentrations of microplankton (A), nanoplankton (B) and picoplankton (C) at intake and outlet of Wolsong NPP from July 2006 to June 2008.
The chlorophyll a concentrations of nanoplankton ranged from 0.02 μg L-1 to 0.90 (mean 0.26) μg L-1 at intake and from 0.02 μg L-1 to 0.60 (mean 0.16) μg L-1 at outlet (Fig. 6B). The amount of mean decrease in chlorophyll a concentrations was 0.10 μg L-1. So the FR of chlorophyll a concentrations of nanoplankton was 0.0~66.7 (mean 38.5)% (Fig. 7A). They were markedly reduced in August (63.6%) and October (66.7%) 2006, and July 2007 (65.5%). But, there was little difference in FR between intake and outlet in June 2006 (5.0%), December 2007 (9.5%), and March 2008 (0.0%) (Fig. 7).
On the other hand, the chlorophyll a concentrations of picoplankton ranged from 0.11 μg L-1 to 1.32 (mean 0.42) μg L-1 at intake and from 0.05 μg L-1 to 0.56 (mean 0.26) μg L-1 at outlet (Fig. 6C). The amount of mean decrease in chlorophyll a concentrations was 0.22 μg L-1. So the FR of chlorophyll a concentrations of picplankton was 9.1~83.3 (mean 52.4)% (Fig. 7). They were markedly reduced in July (83.3%), October 2006 (75.0%), and January (61.8%), March (66.7%) 2007. But, there was little difference in FR between intake and outlet in February 2008 (9.1%) (Fig. 7A).
Accordingly, the mean decrement in total chlorophyll a concentrations was 54.4%. The mean decrement of chlorophyll a concentrations of microplankton was at 58.9% and higher than that of nanoplankton (38.5%) and picoplankton (52.4%). These results showed that the entrainment effects on microplankton by PTCS were higher than those of nanoplankton and picoplankton. The seasonal changes in FR of total chlorophyll a concentrations ranged from 36.4% (in winter season) to 62.8% (in summer season) (Fig. 7B). The FR of chlorophyll a concentrations of microplankton, nanoplankton and picoplankton ranged from 29.3% to 52.3%, ranged from 33.0% to 59.0% and ranged from 34.1% to 65.1%, respectively. Our results showed that the entrainment effects on chlorophyll a concentrations were the highest in summer when the ambient seawater temperature was high (>20oC) (Fig. 7B).
5. FR of the carbon assimilation number
The carbon assimilation number of phy toplankton ranged from 2.6 to 48.1 (mean 14.4) mgC chl a-1 hr-1 at intake and from 1.5 to 30.6 (mean 6.1) mgC chl a-1 hr-1 at outlet during the survey periods (Fig. 8). The FR of carbon assimilation number of hytoplankton was 11.8~91.6 (mean 57.6%) (Fig. 9A). They were markedly reductions in July (73.5%), and September (91.6%) 2006, June (74.0%) and July (79.3%) 2007, and June 2008 (75.1%). So the seasonal changes in FR of carbon assimilation number were 50.6%, 47.5%, 76.8% and 42.9% in winter, spring, summer and autumn, respectively (Fig. 9B). Our results showed that the entrainment effects on carbon assimilation number were the highest in summer.
Fig. 8. Changes in carbon assimilation number of phytoplankton at intake and outlet of Wolsong NPP during July 2006~June 2008.
Fig. 9. The yearly (A) and seasonal (B) changes in FR of carbon assimilation number by PTCS of Wolsong NPP from July 2006 to June 2008.
DISCUSSION
The mean decrement of standing crops of total phytoplankton, microplankton, and nanoplankton by PTCS was 27.0%, 34.1% and 12.4%, respectively. The mean decrement of standing crops of diatoms and dinoflagellates was 33.9% and 29.7%, respectively. The decrease of diatoms abundances by PTCS was higher than those of dinoflagellates. So, the diatoms are very sensitive to temperature rise in comparison with members of Dinophyceae and Chlorophyceae. Therefore, it is considered that the sudden temperature rise may have a strong effect on diatoms (Hirayama and Hirano 1970; Carpenter 1973).
In this study, the diatom C. debilis, C. socialis, L. danicus, P. seriata, S. costatum-like species, T. decipiens and dinoflagellate Prorocemtrum sp. were major species. Among them, the decrement of the standing crops of C. socialis by PTCS was higher than that of other species, but the decrement of T. decipiens was lower than that of other species. Hirayama and Hirano (1970) reported that the growth of S. costatum was affected by high temperature at 35oC, and is completely damaged by exposure to 37oC, whereas Chlamydomonas sp. was affected by high temperature at 43oC, in laboratory experiments. S. costatum, therefore, was more sensitive to high temperature than Chlamydomonas sp. They concluded that high temperature and residual chlorine effluents from a power plant discharging into the open sea, should not cause great damage to marine phytoplankton. Among the major species, the decrement of standing crops of C. socialis was higher than that of other species. The standing crops of major species by PTCS, however, were reduced or increased irregularly, according to survey time. Our results showed that all the phytoplankton species, including the major species which were observed generally throughout the year, by PTCS clearly were affected. FR them, by the way, after PTCS was variable. These results indicated that the all phytoplankton species responded differently to operation conditions of power plant such as elevated temperature, chlorination, and mechanical impacts.
The mean decrement of chlorophyll a concentrations of size-fractionated class (microplankton, nanoplankton and picoplankton) by PTCS was 58.9%, 38.5% and 52.4%, respectively. Our results showed that the microplankton (>20 μm) were more sensitive than nanoplankton (5~20 μm) and picoplankton (<5 μm). The seasonal variations of the mean decrement of chlorophyll a concentrations were 36.4~ 62.6%. The highest value of decrement was observed in summer when the ambient temperature of seawater was high but the lowest value of it was observed in winter.
The comparisons of the entrainment effects on phytoplankton by PTCS at power plants in variable sites are in Table 2. Fox and Moyer (1973) reported that the decrement of chlorophyll a concentrations at Florida power plant was variable. Takesue and Tsuruta (1978) reported that the decrement of chlorophyll a concentrations at Owase-Mita power plant was 71.0~77.0% in August and 31.0~46.0% in January. KEPCO (2010) reported that the decrement of chlorophyll a concentrations at Younggwang nuclear power plant was at a mean of 33.6%. The decrement of phytoplankton carbon assimilation number was 11.8~91.6 (mean 57.6%). The seasonal variations of it were 42.9 (in winter)~76.8% (in summer). So, there were marked reductions of carbon assimilation number in summer. Hamilton et al. (1970) showed that increased water temperature as having an effect on the marine organisms present. Effects are most profound immediately following heat exposure and their severity seems to be proportional to the temperature of the intake water. He reported the decrease of primary productivity was 50.5~91.3% at Chalk point, USA. Fox and Moyer (1975) reported the primary productivity dropped an average of 25.9% at Florida power plant. Flemer and Sherk Jr. (1977) reported that photosynthetic rates didn’t change at 18~19oC but dropped an average 58% at 26oC in Vienna stream station. Takesue and Tsuruta (1978) reported that the depression of photosynthetic activity at Owase-Mita power plant was 71~77% in August and 31~46% in January. Yeo and Kim (1998) reported that the decrease of chlorophyll a concentrations after entrainment was 20.8~59.0 (mean 37.1%) in Kori nuclear power plant and was 15.5~34.9 (mean 25.0%) in Seocheon thermal plant. Choi et al. (2004) analyzed on the pigment composition of phytoplankton assemblages using HPLC at four NPPs in Korea in April 2004 and they reported the concentrations of chlorophyll a decreased 9.7% and 86.5% in Younggwang and Wolsong NPP, respectively but increased to 130.3% and 85.0% in Kori and Ulchin NPP, respectively. They, therefore, indicated that the entrainment effects on the biomass of phytoplankton varied considerably and were site-specific. KEPCO (2010) reported that the primary productivity of phytoplankton dropped an average of 32.1% at Younggwang NPP. Previous studies showed that the entrainment effects on phytoplankton communities were highly variable because the phytoplankton species responded differently to various parameters such as elevated temperature, chlorination, and mechanical impact.
Table 2. Comparisons of the entrainment effects on phytoplankton by PTCS of power plant in variable sites
The sampling effort required to measure effects to statistically acceptable criterion in receiving waters is immense. In a detailed study at the Millstone power station, it was found that although 80~100% of the primary productivity was suppressed during PTCS, no significant differences could be detected between mixing zone and control areas. The authors concluded that 88 samples were required at each station to detect a plus or minus 5% change in productivity. Detecting a 10% change would require 22 samples at each station, a 20% change in six samples. The general interpretation of field data on phytoplankton and comparisons of different site studies require some caution. Three main problems arise: (1) differences in sampling methods and locations in relation to the design and configuration of the cooling system. Sampling difficulties become formidable when the problem of power plant effects is viewed in terms of field studies. In this regard, Carpenter et al. (1974) considered the effect of ‘patchiness’ in the vicinity of a power plant and determined the number of samples required for a given level of precision. Other considerations that effect evaluation of baseline information in power plant studies are the variable distribution from year to year of the phytoplankton biomass and species composition; (2) differences in the methods of incubation and temperatures; and (3) whether the sampling intensity is adequate for correct statistical treatment, particularly for separating out such factors as spatial and temporal heterogeneity (Langford 1990).
In the cooling system of a thermal/nuclear power plant, phytoplankton would severely be damaged both by temperature rise in the condenser and by mechanical destruction and by chlorination. When they are subjected to heat shock alone, the impairment of photosynthesis would be temporary and the recovery would take place. In the thermal effluent water, however, the additional factors of mechanical stress and chlorination cannot be overlooked. Harmful effects of the cooling system on phytoplankton seem not always to be temporary. We point out the effect of mechanical stress and chlorination shock on phytoplankton and the recovery of photosynthetic activity by passage through the cooling systems are subjects for further detailed study.
Reference

2.Brook AJ and AL Baker. 1972. Chlorination at power plants: impact on phytoplankton productivity. Science 176:1414-1415.


3.Carpenter EJ, BB Peck and SJ Anderson. 1972. Cooling water chlorination and productivity of entrained phytoplankton. Mar. Biol. 16:37-40.

4.Carpenter EJ. 1973. Brackish-water phytoplankton response to temperature elevation. Est. Coastal Mar. Sci. 1:37-44.

5.Carpenter EJ, SJ Anderson and BB Peck. 1974. Copepod and chlorophyll-a concentration in receiving water of a nuclear power station and problems associated with their measurements. Est. Coastal Mar. Sci. 2:83-88.

6.Choi HC, YS Kang, JK Choi, TY Song and MH Yoo. 2004. Analysis on the pigment composition of phytoplankton assemblages using HPLC (High Performance Liquid Chromatography) in the adjacent waters of nuclear power plants in spring. J. Korean Soc. Oceanogr. 39:234-242.
7.Dunstall TG. 1985. Effects of entrainment on phytoplankton primary production at four thermal electric generating stations on the Laurentian Great Lakes. Rev. Gesamten. Hydrobiol. 70:247-257.

8.Flemer D and JA Sherk Jr. 1977. The effects of steam electric station operation on entrained phytoplankton. Hydrobiologia 55:33-44.

9.Fox JL and MS Moyer. 1973. Some effect of power plant chlorination on marine microbiota. Chesap. Sci. 14:1-10.

10.Fox JL and MS Moyer. 1975. Effect of power plant chlorination on estuarine productivity. Chesap. Sci. 16:66-68.

11.Goldman JC and HL Quinby. 1979. Phytoplankton recovery after power plant entrainment. J. Water Poll. Control Fed. 51:1818-1823.
12.Hamilton DA Jr., DA Flemer, CV Keefe and JA Mihursky. 1970. Power plants: effects of chlorination on estuarine primary production. Science 169:197-198.

13.Hirayama K and R Hirano. 1970. Influence of high temperature and residual chlorine on marine phytoplankton. Mar. Biol. 7:205-213.

14.Kang YS. 2008. Characteristics of phytoplankton communities in the coastal waters of power plant. Algae 23:31-52.

15.Kang YS and JK Choi. 2001. Ecological characteristics of phytoplankton communities in the coastal waters of Gori, Wulseong, Uljin and Youngkwang I. Species composition and distritution (1992~1996). Algae 16:85-111.
16.Kang YS and JK Choi. 2002. Ecological characteristics of phytoplankton communities in the coastal waters of Gori, Wulseong, Uljin and Youngkwang II. Distributions of standing crops and envrionmental variables (1992~1996). J. Korean Soc. Oceanogr. 26:77-82.
17.Kang YS, JK Choi and HM Eum. 2003. Ecological characteristics of phytoplankton communities in the coastal waters of Gori, Wulseong, Uljin and Youngkwang III. Distribution of dominat species and environmental variables. Algae 18: 29-47.

18.KEPCO (Korea Electric Power Corporation). 2010. Environmental survey and assessment around the Younggwang nuclear power plant (2007~2009). 405p.
19.Langford TEL. 1990. Ecological effects of thermal discharges. London: Elsevier Applied Science. 468p.
20.Lee JH and EH Lee. 1997. Seasonal varinations of phytoplankton communities in the vicinity of thermal power plants (Boryung and Seochon). Algae 12:105-115.
21.Morgan RP and RD Stross. 1969. Destruction of phytoplankton in the cooling water supply of a steam electric station. Chesap. Sci. 10:165-171.

22.Parsons TR, Y Maita and CM Lalli. 1984. A manual of chemical and biological methods for seawater anaylysis. Pergamon Press. 173p.
23.Suresh K, MS Ahamed, G Durairaj and KVK Nair. 1995. Environmental physiology of the mole crab Emerita asiatica, at a power plant discharge area on the east coast of India. Environ. Pollut. 17:161-165.
24.Takesue K and A Tsuruta. 1978. The thermal effects of cooling system of a thermal power plant on photosynthesis of marine phytoplankton. J. Oceanogr. Soc. Japan 34:295-302.

25.Warinner JB and ML Brehmer. 1966. The effects of thermal effluents on marine organisms. Int. J. Air Wat. Pollut. 10: 277-289.
26.Yeo HG and JH Shim. 1992. Ecological effects of thermal effluents in the Korean coastal waters III. Abiotic environmental and the community structure of primary producers in the vicinity of Kori nuclear power plant. Korean J. Environ. Biol. 11:124-130.
27.Yeo HG and MK Kim. 1998. The effect of phytoplankton passed through the cooling systems of nuclear power plant and thermoelectric power plant. Korean J. Environ. Biol. 16:101-105.
28.Yi SK and P Chin. 1987. Effects of cooling water system of power plant on marine organisms I. Effects on primary production. Bull. Korean Fish. Soc. 20:381-390.
Vol. 40 No. 4 (2022.12)
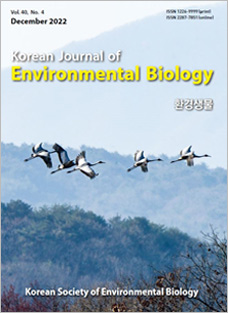
Frequency quarterly
Doi Prefix 10.11626/KJEB.
Year of Launching 1983
Publisher Korean Society of Environmental Biology




Online Submission
submission.koseb.org
KOSEB
Korean Society of Environmental Biology
Contact info
Any inquiries concerning Journal (all manuscripts,
reviews, and notes) should be addressed to the managing
editor of the Korean Society of Environmental Biology.
Yongeun Kim,
Korea University, Seoul 02841, Korea.
E-mail: kyezzz@korea.ac.kr /
Tel: +82-2-3290-3496 / +82-10-9516-1611