Journal Search Engine
ISSN : 2287-7851(Online)
DOI : https://doi.org/10.11626/KJEB.2013.31.4.376
[6]-Gingerol Attenuates Radiation-induced Cytotoxicity and Oxidative Stress in HepG2 Cells
Abstract
- 0053-01-0031-0004-15.pdf206.8KB
INTRODUCTION
Ionizing radiation (IR) has many practical applications such as medicine, food, agriculture, environment and industry (Chmielewski and Haji-Saeid 2004; Ryan 2012). However, high exposure of IR is associated with harmful effects on human health (Moulder 2004). IR interacts with biomolecules (DNA, RNA, protein, lipid and carbohydrate) and produces toxic free radicals leading to oxidative stress, imbalance state between prooxidant and antioxidant (Held 1997; Curtin et al. 2002; James et al. 2002; Bak et al. 2012). IR-induced oxidative stress may cause cell damage including genomic alterations, lipid peroxidation, mutation and cell death (Riley 1994; Devsagayam et al. 2004). In order to regulate the oxidative stress, there are endogenous antioxidant defense systems which includes enzymatic antioxidants (superoxide dismutase, catalase, glutathione peroxidase, etc.) and non-enzymatic antioxidants (glutathione, ascorbate, bilirubin, α-tocophenrol etc.) (Kunwar and Priyadarsini 2011). Even if cells have many endogenous antioxidants, these systems may not protect cell against IR-induced oxidative stress. Therefore, it is necessary to supplement exogenous antioxidant under oxidative stress condition (Hosseinimehr 2007). In regard with radioprotector, polyphenol in dietary plant is of considerable interest due to potent antioxidant activity (Weiss and Landauer 2003).
Ginger has been widely used as medicine (common cold, fever, digestive order and arthritis) and food (flavoring agent, spice, condiment, beverage and tea) for a long time (White 2007). It contains bioactive polyphenols such as gingerol, shogaol, paradol and zingerone (Shukla and Singh 2007). In particular, [6]-gingerol has been reported to have many phar-macological effects (Kim et al. 2005; Nigam et al. 2009; Lee et al. 2011). [6]-gingerol (structure shown in Fig. 1) is a major non-volatile pungent compound in the rhizome of ginger (Zingiber officinale). It is known to have various biological effects such as antioxidant, anti-inflammatory and anticancer properties (Dugasnai et al. 2010; Lv et al. 2012). However, there is no report on the radioprotective effects of [6]- gingerol in HepG2 cells.
Fig. 1. Structure of [6]-gingerol.
The purpose of this study is to investigate the harmful effects of HepG2 cells after exposure of IR and the protective effects of [6]-gingerol against IR-induced cytotoxicity and oxidative stress (antioxidant enzyme status) in cultured HepG2 cells.
MATERIALS AND METHODS
1. Materials
[6]-gingerol (CAS No. 23513-14-6) was purchased from Sigma Chemical Co. (St. Louis, MO, USA). Dulbecco’s modified Eagle’s medium (DMEM) was purchased from Welgene Inc. (South Korea). Fetal Bovine Serum (FBS), Phosphate Buffered Saline (PBS) were purchased from Thermo Fisher Scientific Inc. (Waltham, MA, USA). Antibiotic (10,000 U mL-1 of penicillin, 10,000 μg mL-1 of streptomycin and 25 μg mL-1 of amphotericin B) and trypsin EDTA was obtained from Life Technologies Corporation (CA, USA). Cell proliferation kit I for MTT assay was purchased from Roche Applied Science (IN, USA). Superoxide dismutase (SOD) and catalase (CAT) assay kit were obtained from Cayman Chemical Company (MI, USA).
2. Cell culture and irradiation
Human hepatoblastoma HepG2 cells were cultured and maintained in DMEM supplemented with 10% FBS and 1% penicillin-streptomycin antibiotic in a humidified 5% CO2 incubator at 37℃. The medium was changed every 2~3 days and cells were plated at an appropriate density according to each experimental scale. Cells were irradiated (dose rate: 0, 1, 5 and 10 Gy) using 60Co gamma cell irradiator (AECL, Canada) in Korea Atomic Energy Research Institute (Jeoungeup, Korea).
3. MTT assay
HepG2 cells were seeded at the density of 1×104 cells in each well of the 96 well plates and incubated for 24 h at 37℃. [6]-gingerol was added to cell in fresh media at 5, 10, 20, 40 and 80 μM concentration. Cells were incubated for next 24 h. Cell viability was measured by the MTT assay as described previously (Mosmann et al. 1983). Cells were treated with MTT solution (5 mg mL-1 in Phosphate Buffered Saline) and incubated for 4 h at 37℃, yellowish crystal formed. The crystal was solubilized by adding solubilization solution (10% SDS in 0.01 M HCl) and incubated for overnight at 37℃. The absorbance was read at 570 nm. The experiments were performed at least three times.
4. Trypan Blue assay
Cell viability was measured by trypan blue assay previously described (Jauregui et al. 1981). Cells were seeded at the density of 1×106 cells in each cultured plate. After 1 day incubation, cells were subjected to irradiate with different doses (0, 1, 5, and 10 Gy). Cells were incubated for different time period at 0, 1, 2 and 3 days post-irradiation. After incubation for different time period, cells were removed by trypsinization. Cell suspension (200 μL) was mixed with 0.4% trypan blue solution (800 μL) and the mixture were incubated for 5 min at room temperature. Cells were counted using a dual-chamer hemocytometer and a light microscope. The percentage of cell viability (untreated control cells) was calculated as 100%. The experiments were performed at least three times.
5. Estimation of Superoxide Dismutase (SOD) activity
The activity of SOD was performed according to the manufacturer’s instruction (Cayman Chemical Company, USA). Briefly, cells were collected by using rubber policeman and homogenized by using sonicator in 1~2 mL of cold 20mM HEPES buffer containing 1 mM EGTA, 210 mM mannitol and 70 mM sucrose, pH 7.2 and centrifuged at 12,000×g for 10 min at 4℃. The supernatants were collected for assay and stored on ice. Protein concentration was determined by Bradford method (Bradford 1976). One unit of enzyme activity was defined by the 50% dismutation of superoxide radical. In a 96 well plate, 200 μL of tetrazolium salt was added in different well for each sample and subsequently added 10μL of sample in each well. The reaction was initiated by adding 20 μL of xanthine oxidase and the plate was incubated on a shaker for 20 min at room temperature. The absorbance was measured at 450 nm by using plate reader. The experiments were performed at least three times.
6. Estimation of Catalase (CAT) activity
The activity of CAT was performed according to the manufacturer’s instruction (Cayman Chemical Company, USA). The decomposition of H2O2 in presence of CAT was followed by the decrease in absorbance measured by spectrophotometer. Cells were collected by using rubber policeman and homogenized by using sonicator in 1~2 mL of cold 50mM potassium phosphate buffer, pH 7.0, containing 1mM EDTA and centrifuged at 12,000×g for 10 min at 4℃. The supernatants were used for the assay and stored on ice. Protein concentration was determined by Bradford method (Bradford 1976). In a 96 well plate, 20μL of sample was taken to each well and then added 100μL of 100 mM potassium phosphate, pH 7.0 and 30 μL of methanol. The reaction was initiated by adding 20 μL of hydrogen peroxide to each well and incubated on a shaker for 20 min at room temperature. To terminate the reaction, 30 μL of potassium hydroxide was added to the each well and then added 30 μL of catalase purpald as quickly as possible. The plate was incubated for 10 min at room temperature on the shaker. Finally, 10μL of catalase potassium periodate was added to each well and incubated for 5 min at room temperature on the shaker. The absorbance was taken at 540 nm by using a plate reader. The experiments were performed at least three times.
RESULTS
1. Radioprotective effect of [6]-gingerol against IR-induced cytotoxicity
To investigate the cytotoxic effect of [6]-gingerol on HepG2 cells, cell viability was performed by MTT assay. Cells were treated with different concentration (0, 5, 10, 20, 40 and 80 μM) and then incubated for 1 day. As shown in Fig. 2, [6]-gingerol with 5μM concentration did not decrease cell viability. Thus, [6]-gingerol was used as potential radioprotective agent in this study.
Fig. 2. Cell cytotoxicity of [6]-gingerol by using MTT assay. Data are represented as mean±SD (n=3). *p⁄0.05, **p⁄0.01 compared with control group.
To examine the harmful effect of IR in HepG2 cells, cells were irradiated with 0, 1, 5 and 10 Gy. The IR-treated group was incubated for different time (0, 1, 2 and 3 days) to monitor cell morphological change and cell cytotoxicity. As shown in Fig. 3, cell morphology was changed in a time-dependent manner after exposure of IR with 5 Gy. After the different incubation days, cell viability was examined by trypan blue assay (data not shown). Cell viability was used as an indicator of cytotoxicity. As shown in Fig. 4(A), the IR-treated group was decreased the percentage of cell viability in a dose-dependent manner for 3 days after irradiation, with half lethal dose (LD50) of 5 Gy.
Fig. 3. Radiation-induced cell morphological change of HepG2 cells. Cells were exposed to 5 Gy. A: 0 h after IR exposure, B: 24 h after IR exposure, C: 48 h after IR exposure, D: 72 h after IR exposure.
Fig. 4. Radiation-induced cell cytotoxicity (A) and radioprotective effect of [6]-gingerol (B) in HepG2 cells. Cells were incubated for 3 days after irradiation. Data are represented as mean±SD (n=3). *p⁄0.05, **p⁄0.01 compared with control group.
To determine the radioprotective effect of [6]-gingerol against IR-induced cytotoxicity in HepG2 cells, cells were pretreated with or without 5μM [6]-gingerol for 1 h and then irradiated with 0 or 5 Gy. As shown in Fig. 4(B), [6]-gingerol plus IR-treated group was increased the percentage of cell viability as compared to that of IR-treated group. The results suggest that [6]-gingerol could have protective effect against cell cytotoxicity induced by IR.
2. Radioprotective effect of [6]-gingerol against IR-induced oxidative stress
To assess the radioprotective effect of [6]-gingerol against IR-induced oxidative stress in HepG2 cells, antioxidant enzyme activity was examined by the biochemical kit (SOD and CAT activity) assay. As shown in Figs. 5 and 6, IR-treated group was increased both SOD and CAT activity in a dosedependent manner. In contrast, [6]-gingerol plus IR-treated group was suppressed both SOD and CAT activity as compared to that of IR-treated group. It indicates that [6]-gingerol pretreatment could protect cellular oxidative stress induced by IR.
Fig. 5. Radiation-induced SOD activity change (A) and radioprotective effect of [6]-gingerol (B) in HepG2 cells. Data are represented as mean±SD (n=3). *p⁄0.05, **p⁄0.01 compared with control group.
Fig. 6. Radiation-induced CAT activity change (A) and radioprotective effect of [6]-gingerol (B) in HepG2 cells. Data are represented as mean±SD (n=3). *p⁄0.05, **p⁄0.01 compared with control group.
DISCUSSION
There is considerable interest in the radioprotective effects of natural antioxidants against oxidative stress. Some of dietary polyphenols have very potent antioxidant and free radical scavenging activity. They can inhibit cellular genotoxicity, prevent cellular oxidative stress and cytotoxicity (Duthie et al. 1997; Noroozi et al. 1998; Lipkin et al. 1999). Some reports demonstrated that [6]-gingerol, a pungent phenolic compound with a high antioxidant activity, exhibited a variety of biological effects. Kim et al. (2007) reported that [6]-gingerol treatment has been shown to reduce UVB-induced cell damage by reducing reactive oxygen species (ROS) levels and activating caspases-3, -8, -9, cyclooxygenase-2 (COX-2) and Fas expression in human keratinocytes cell line (HaCat). Some researchers reported that [6]-gingerol exhibited anti-cancer effects (Shukla et al. 2007). Chakraborty et al. (2012) reported that [6]-gingerol had anti-oxidative property and protective effect against arsenic-induced toxicity by improving impaired insulin signaling pathway. In this study, we reported that [6]-gingerol, natural antioxidant, protects HepG2 cells against IR-induced cell damage by reducing cell viability and an increased level of SOD and CAT activity.
Oxidative stress represents imbalance of the pro-oxidants and antioxidants in cell. IR is known to induce oxidative stress via generation of ROS which can lead to cell death. The cellular antioxidant defense system plays an important role in protecting cell against oxidative stress. In fact, changes in the activity of antioxidant enzymes can be regarded as a biomarker. There are major antioxidants in cells such as SOD, CAT and glutathione (GSH). SOD catalyzed the dismutation of O2- to H2O2 and CAT converts H2O2 into water. In this study, HepG2 cells with exposure of IR evoked significant changes of the activity of SOD and CAT. Both activities were increased after exposure of IR, indicating that the fact was the activation of antioxidant defense system in HepG2 cells. Alia et al. (2006) reported that quercetin protects HepG2 cells against t-BOOH-induced oxidative stress by reducing SOD and CAT activity. In regard with oxidative stress, our data about change of SOD and CAT activity was in agreement with the results obtained Alia et al. despite different stress factor.
In conclusions, we reported the protective effect of [6]- gingerol against IR-induced cytotoxicity and oxidative stress in HepG2 cells at the first time. Following irradiation with 0 to 10 Gy, IR induced cytotoxicity and oxidative stress in a dose-dependent manner in HepG2 cells. The LD50 of HepG2 cells was about 5 Gy. Pretreatment of 5 μM [6]-gingerol attenuated IR-induced cell viability loss and an increased level of antioxidant enzymes (SOD and CAT) activity. These findings suggest that [6]-gingerol could be useful as a radioprotector.
ACKNOWLEDGEMENTS
This study has been done under the Creative Research Program by the Ministry of Science, ICT and Future Planning (MSIP) of Korea.
Reference

2.Bak MJ, M Jun and WS Jeong. 2012. Antioxidant and Hepatoprotective effects of the red ginseng essential oil in H2O2- treated HepG2 cells and CCl4-treated mice. Int. J. Mol. Sci. 12:2314-2330.

3.Bradford MM. 1976. Rapid and sensitive method for the quantification of microgram quantities of protein utilizing the principle of protein-dye binding. Anal. Biochem. 72:248- 254.

4.Chakraborty D, A Mukherjee, S Sikdar, A Paul, S Ghosh and AR Khuda-Bukhsh. 2012. [6]-Gingerol isolated from ginger attenuates sodium arsenite induced oxidative stress and plays a corrective role in improving insulin signaling in mice. Toxicol. Lett. 210:34-43.

5.Chmielewski AG and MH Saeid. 2004. Radiation technologies: past, present and future. Radiat. Phys. Chem. 71:16-20.

6.Curtin JF, M Donovan and TG Cotter. 2002. Regulation and measurement of oxidative stress in apoptosis. J. Immunol. Methods 265:49-72.

7.Devasagayam TPA, JC Tilak, KK Boloor, KS Sane, SS Ghaskadbi and RD Lele. 2004. Free radical and Antioxidants in human health: Current status and future prospects. JAPI 52:794-804.
8.Dugasani S, MR Pichika, VD Nadarajah, MK Balijepalli, S Tandra and JN Korlakunta. 2010. Comparative antioxidant and anti-inflammatory effects of [6]-Gingerol, [8]-Gingerol, [10]-Gingerol and [6]-Shogaol. J. Ethnopharmacol. 127:515- 520.

9.Duthie SJ, W Johnson and VL Dobson. 1997. The effect of dietary flavonoids on DNA damage (strand breaks and oxidized pyrimidines) and growth in human cells. Mutat. Res. 390: 141-151.

10.Held KD. 1997. Radiation induced apoptosis and its relationship to loss of clonogenic survival. Apoptosis 2:265-282.

11.Hosseinimehr SJ. 2007. Foundation review: Trends in the development of radioprotective agents. Drug Discovery Today. 12:794-805.

12.Jauregui HO, NT Hayner, JL Driscoll, W Holland, MH Lipsky and PM Galletti. 1981. Trypan blue dye uptake and lactate dehydrogenase in adult rat hepatocytes freshly isolated cells, cell suspensions, and primary monolayer cultures. In vitro 17:1100-1110.

13.Kim EC, JK Min, TY Kim, SJ Lee, HO Yang, S Han, YM Kim and YG Kwon. 2005. [6]-Gingerol, a pungent ingredient of ginger, inhibits angiogenesis in vitro and in vivo. Biochem. Biophys. Res. Commun. 335:300-308.

14.Kim JK, Y Kim, KM Na, YJ Surh and TY Kim. 2007. 6-Gingerol prevents UVB-induced ROS production and COX-2 expression in vitro and in vivo. Free Radic. Res. 41:603- 614.

15.Kunwar A and KI Priyadarsini. 2011. Free radicals, oxidative stress and importance of antioxidants in human health. J. Med. Allied Sci. 1:53-60.
16.Lee C, GH Park, CY Kim and JH Jang. 2011. [6]-Gingerol attenuates β-amyloid induced oxidative cell death via fortifying cellular antioxidant defense system. Food Chem. Toxicol. 49:1261-1269.

17.Lipkin M, B Reddy, H Newmark and SA Lamprecht. 1999. Dietary factors in human colorectal cancer. Annu. Rev. Nutr. 19:545-586.

18.Lv L, H Chen, D Soroka, X Chen, TC Leung and S Sang. 2012. 6-Gingerdiols as the major metabolites of 6-Gingerol in cancer cells and in mice and their cytotoxic effects on human cancer cells. J. Agric. Food Chem. 60:11372-11377.

19.Mosmann T. 1983. Rapid colorimetric assay for cellular growth and survival: application to proliferation and cytotoxicity assays. J. Immunol. Methods 65:55-63.

20.Moulder JE. 2004. Post irradiation approaches to treatment of radiation injuries in the context of radiological terrorism and radiation accidents: a review. Int. J. Radiat. Biol. 80:3- 10.

21.Nigam N, K Bhui, S Prasad, J George and Y Shukla. 2009. [6]- Gingerol induces reactive oxygen species regulated mitochondrial cell death pathway in human epidermoid carcinoma A431 cells. Chem. Biol. Interact. 181:77-84.

22.Noroozi M, WJ Angerson and MEJ Lean. 1998. Effects of flavonoids and vitamin-C on oxidative DNA damage to human lymphocytes. Am. J. Clin. Nutr. 67:1210-1218.
23.Riley PA. 1994. Free radicals in biology: oxidative stress and effects of ionizing radiation. Int. J. Radiat. Biol. 65:27-33.

24.Ryan JL. 2012. Ionizing radiation: The good, the bad and the ugly. J. Invest. Dermatol. 132:985-993.

25.Shukla Y and M Singh. 2007. Cancer preventive properties of ginger: A brief review. Food Chem. Toxicol. 45:683-690.

26.Shukla Y, S Prasad, C Tripathi, M Singh, J George and N Kalra. 2007. In vitro and in vivo modulation of testosterone mediated alterations in apoptosis related proteins by [6]-gingerol. Mol. Nutr. Food Res. 51:1492-1502.

27.Weiss JF and MR Landauer. 2003. Protection against ionizing radiation by antioxidant nutrients and phytochemicals. Toxicology 189:1-20.

28.White B. 2007. Ginger: An overview. American Family Physician 75:1690-1691.
Vol. 40 No. 4 (2022.12)
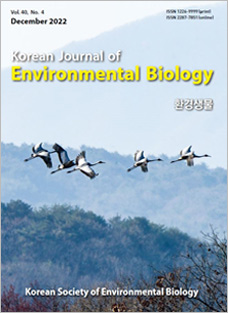
Frequency quarterly
Doi Prefix 10.11626/KJEB.
Year of Launching 1983
Publisher Korean Society of Environmental Biology




Online Submission
submission.koseb.org
KOSEB
Korean Society of Environmental Biology
Contact info
Any inquiries concerning Journal (all manuscripts,
reviews, and notes) should be addressed to the managing
editor of the Korean Society of Environmental Biology.
Yongeun Kim,
Korea University, Seoul 02841, Korea.
E-mail: kyezzz@korea.ac.kr /
Tel: +82-2-3290-3496 / +82-10-9516-1611